Since the COVID-19 pandemic we constantly hear mRNA-, viral vector-, DNA-, protein-, killed- and, live attenuated- based vaccines. What is the difference? They are just different types of vaccine formulations, all with the same end-result, to stimulate an immune response and protect against a specific disease.
History of vaccines: The first attempts to prevent infectious disease was in 1000AD in China, where crude contents of smallpox vesicles were used to inject healthy individuals. Fatalities were uncommon in the individuals inoculated with the smallpox vesicles.
Fast forward to the 1700’s and Edward Jenner noted that milkmaids, who had contracted cowpox (cow version of human smallpox), were protected against smallpox. So, in the late 1790s, Jenner undertook protective tests where he immunised people with cowpox and were found to be protected against smallpox. This method drew much criticism by the public due to misinformation and myths – fake news. However, the method became popular from the 19th century onwards. It finally led to mass global-vaccinations and resulted in the eradication of smallpox from the world in a decade of 1967-1977. An amazing achievement.
Live (attenuated) vaccines: This type of vaccine contains a weakened (attenuated) form of the pathogen (virus or bacteria) that causes the disease. Whilst the attenuated pathogen can still replicate within the body, it does not cause disease. It induces strong immune responses, such as antibodies against the pathogen itself, leading to immunity and protection against disease. Live (attenuated) vaccines can stimulate a robust and tough immune response, and often creates lifelong immunity and protection. Such vaccine formulation mimics that of natural infection. However, they may pose risk to individuals with weakened immune systems such as those receiving transplants or undergoing chemotherapy. These vaccines require strict cold-chain temperatures to retain their potency, which can be challenging in resource limited countries. Examples of such vaccines, include the measles, mumps, rubella (MMR) vaccine, varicella (chickenpox) vaccine, the oral polio vaccine, dengue, respiratory syncytial virus, zika virus, cholera vaccine, Bacille-Calmette-Guerin (BCG) vaccine against tuberculosis.
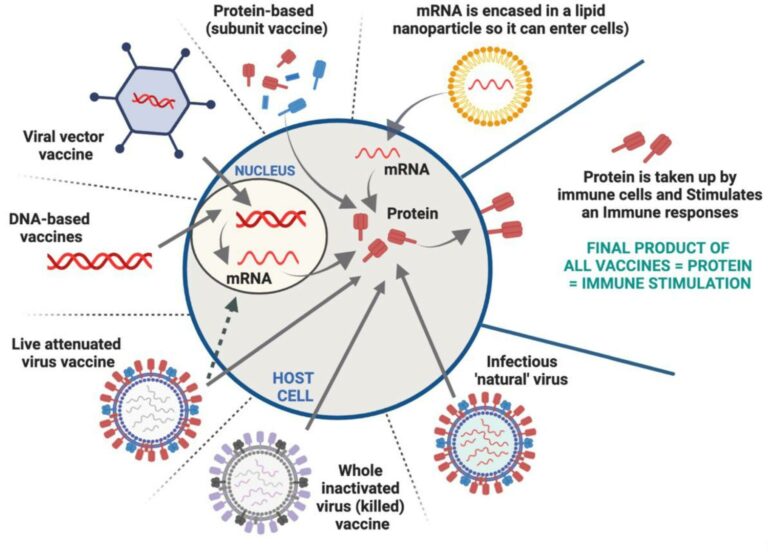
Inactivated (killed, dead) vaccines: To make the vaccines safer from the live-attenuated vaccine method, they destroy the pathogen by killing it, however while still maintaining ability to stimulate immune responses. These vaccines contain pathogens (virus or bacteria) that have been inactivated (killed), either by chemicals (beta-propiolactone or formaldehyde), heat, or radiation. While the inactivated pathogen is no longer able to cause disease, it is still able trigger immune responses. An example of a killed vaccine is against poliomyelitis developed by Salk in 1960, which involved treating the virus with various chemicals. This vaccine had an enormous impact on the incidence of the vaccine before being replaced by the live attenuated vaccine developed by Sabin in 1973. These vaccines are stable and do not require cold temperatures for storage making them easier to store and distribute. They are suitable for immune compromised individuals who may not be able to receive live attenuated vaccine types. Examples of inactivated or killed vaccines include the inactivated polio vaccine (IPV), the hepatitis A vaccine, whooping cough, dengue, and some influenza vaccines. More recently Sinovac CoronaVac vaccine, the Bharat Biotech Covaxin, and the Sinopharm vaccines were developed for COVID-19 and authorised and used in some countries.
Disasters: In spite of the progress in developing vaccines using traditional methods to several key diseases, there were a number of disasters, in particular, the typhoid disaster in 1904, the diphtheria disasters in USA, Austria, Russia, China, Australia in 1928, the Lubeck disaster in 1932 and, the Cutter disaster in 1955, which were a result of inadequate attenuation, contaminations, inadequate preservatives, inadequate heating (killed) pathogens. As a result, these disasters led to improved procedures, safety of vaccine formulations, improved stability, efficacy, costs, and led to regulatory measures to assure proper and improved laboratory conditions, and training of personnel.
Safer and effective vaccines: Public awareness of health and safety is at levels far higher than was 70 years ago. Vaccines must now meet higher standards of safety and biochemical characterisation than they did in the past. Some of the vaccines developed in the past would not even meet the minimum standards required today. Hence, to be successful in this situation, new molecular and biological technologies are required to be used which have become available in the past few decades. Advances in the fields of chemistry, biochemistry, structural biology, immunology, genetics, are now included in the development of new and improved vaccines, in an attempt to move from traditional live virus vaccines to the theoretical ‘safer and highly purified’ but ‘less immunogenic’ vaccines. As such, new improved methods of developing vaccines include different technologies, including, protein, DNA, viral vectors, and mRNA technologies and delivered in an appropriate formulation to stimulate immune responses. Such vaccines, however, require multiple doses or booster shots to achieve optimal immunity.
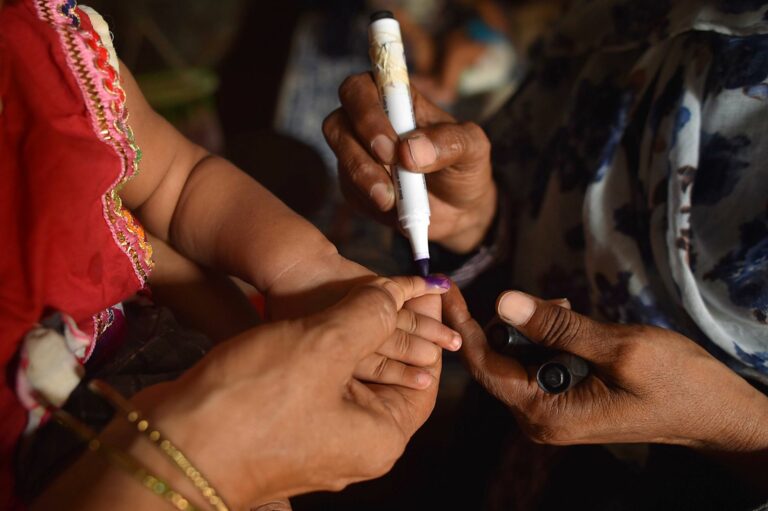
The range of current vaccines
1. Protein-based (subunit) vaccines: Contain highly purified fragments of the infective regions of the pathogen in the form of protein, and are used to stimulate immune responses. These vaccines often require an additional stimulant (adjuvant), which enhances the immune response to the protein. Protein-based vaccines are generally considered safe as they do not contain live pathogens or genetic material. These vaccines are stable and do not require strict cold chain storage, making them easier to distribute and administer. Examples of protein-based vaccines include the hepatitis B vaccine, human papillomavirus (HPV) vaccine assembled as small virus-like particles. Several vaccines against melanoma, HIV, hepatitis B and cancer are in human clinical trials with some showing long term efficacy. One example of a protein-based COVID-19 vaccine is the Novavax vaccine.
2. Viral vector vaccines: The ideal vaccine for many diseases is a live attenuated derivative of the pathogen which can induce protective immunity of the pathogen without causing any side effects. Barriers to the development of such vaccines, however, give rise to difficulties in growing the pathogen in the lab and difficulties in attenuating the pathogen. One strategy of overcoming these barriers is to insert the pathogen’s genes into a non-pathogenic organism. The harmless virus serves as a vehicle (vector) for the expression of the genes coding for sections of the pathogen. The vector delivers genetic material, in the form of mRNA or DNA, of the virus or pathogen of interest into human immune cells, and protein is produced, which then stimulates an immune response against the targeted disease. Different harmless vectors have been used in human clinical trials against several diseases in the last 30 years including vaccinia virus, avipox virus, vesicular stomatitis virus, adenovirus, lipid vectors. More recently, Johnson & Johnson COVID-19 vaccine used a modified adenovirus as the vector and the Oxford-AstraZeneca vaccine used a modified version of a chimpanzee adenovirus.
3. DNA-based vaccines: Immunisation with a small, circular piece of DNA that contains the genetic instructions for producing a specific viral or pathogen protein may hold advantages to use as vaccines, because no infectious agent is involved. In 1990 it was noted that injection of DNA into the muscles of mice, was taken up by cells and expressed as protein. This revolutionised the concept of vaccines as, unlike proteins made in test tubes, DNA was able to be injected and converted to proteins inside living cells, which do not have to go through the expensive purification steps to make proteins. Various methods over the years have been developed to efficiently deliver DNA into cells with several human clinical trials completed for different diseases in the last 25 years with stimulation of immune responses against the targeted disease and good safety profiles. DNA vaccines are quick and easy to be produced and can be stored without the requirement for cold chain storage. INO-4800 vaccine received Emergency use authorisation in certain countries to be used against COVID-19.
4. mRNA-based vaccines: These vaccines utilise a small piece of mRNA that encodes the instructions for making specific viral or pathogen proteins. mRNA is targeted to immune cells and once inside the cells, the mRNA is translated into protein, which stimulates an immune response. mRNA-based vaccines can be developed quickly compared to other vaccines and do not require the cultivation or inactivation of an actual pathogen. Such vaccines can be quickly modified to target new variants or strains of a virus or pathogen and they do not contain live pathogens or use viral vectors, reducing the risk of causing the disease. Over the last few decades several human clinical trials were conducted using such vaccines however further research was required to optimise its efficacy to enter cells. In parallel, nanoparticles were used and optimised in the last 50 years to deliver drugs and used to deliver vaccines. As such, Pfizer-BioNTech and Moderna COVID-19 vaccines, consist of mRNA entrapped into lipid nanoparticles and used as emergency use and full approval in some countries. These vaccines have shown high efficacy in stimulating immune responses and preventing and/or minimising symptoms associated with COVID-19 disease.
Vaccines against non-infectious pathogens
As most vaccines described are against viruses and bacteria, the same concept can be applied to generate vaccines against cancer, autoimmune diseases and even against drug addiction. We have developed such vaccines using protein or peptides (small proteins) with efficacy in animal models and in human clinical trials. The future holds much promise that some of these vaccines will be commercialised to prevent diseases.
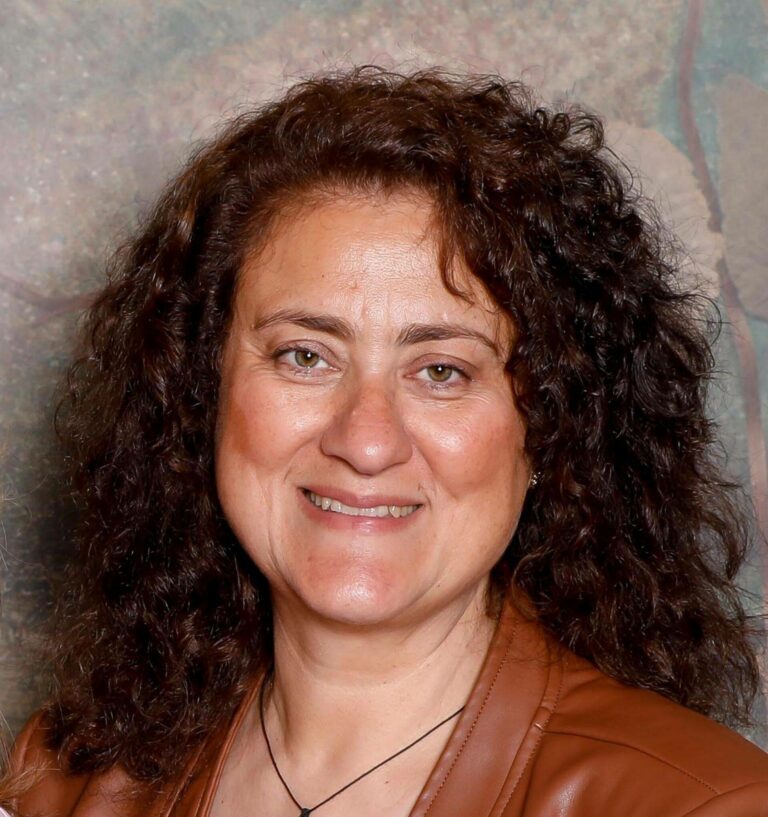
For further information, read publications by Vasso Apostolopoulos by searching on Pubmed or Scopus.